FAQ [Frequently Asked Questions]
- Glossary of Terms
- Leveling / Collimating Using Your Base
- Which Focuser for Me?
- What’s the Big Deal About Focusing?
- Why a Feather Touch?
- Why are Brakes a Good Thing?
- How Much Weight Can My Focuser Support?
- How Long Does My Draw Tube Need To Be?
- Intro to Telescopes, 101
Glossary of Terms, and Other Fascinating Things…
How are Starlight Instruments Focuser Model Numbers Assigned? Our focusers are numbered using four numbers:
— The first two numbers in our model number indicate the minimum clear opening of the drawtube at the entry side of the light cone, in inches. [For example, the 3545 has a clear opening of 3.5 inches.]
— The last set of numbers indicates the travel distance of the draw tube. [For example, the 3545 has 4.5 inches of travel.]
Optical Tube Assembly [OTA]: The Optical Tube Assembly is typically defined to include the mirrors|lenses and their related support systems.
Apochromat [APO]: An apochromat, or apochromatic lens (APO), is a lens that has better color correction than the much more common achromat lenses. Chromatic aberration is the phenomenon of different colors focusing at different distances from a lens. Chromatic aberration produces soft overall images, and color fringing at high-contrast edges, like an edge between black and white. Astronomers face this problem more so with telescopes that use lenses rather than mirrors. Achromatic lenses are corrected to bring two wavelengths (typically red and blue) into focus in the same plane. Apochromatic lenses are designed to bring three wavelengths (typically red, green, and blue) into focus in the same plane.
The residual color error (secondary spectrum) can be up to an order of magnitude less than for an achromatic lens of equivalent aperture and focal length.
Apochromats are also corrected for spherical aberration at two wavelengths, rather than one as in an achromat.
Collimated Light: Collimated Light is light whose rays are nearly parallel, and therefore will spread slowly as it propagates. The word is derived from “collinear” and implies light that does not disperse with distance (ideally), or that will disperse minimally (in reality). A perfectly collimated beam with no divergence cannot be created due to diffraction, but light can be approximately collimated by a number of processes, for instance by means of a collimator. In optics, a collimator may consist of a curved mirror or lens which brings the light to a specific plane of focus. Collimated light is sometimes said to be focused at infinity. Thus as the distance from a point source increases, the spherical wavefronts become flatter and closer to plane waves, which are perfectly collimated.
Collet: A holding device, specifically, a subtype of chuck, that forms a collar around the object to be held and exerts a strong clamping force on the object when it is tightened via a tapered outer collar.
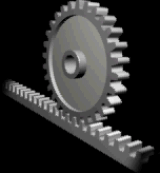
Focuser Housing: This is the body of the focuser
Rack and Pinion Drive: A rack and pinion is a pair of gears which convert rotational motion into linear motion. The circular pinion engages teeth on a flat bar – the rack. Rotational motion applied to the pinion will cause the rack to move to the side, up to the limit of its travel.
Pinion Housing: This is the part that incorporates the Focusing Knobs, Brake and the Fine Focus Reduction Unit and is attached to the Focuser Housing
Rack: This is a linear gear that mates with the pinion. It allows a geared system to translate a rotary motion, from the focus knobs, into a linear motion to the draw tube.
Pinion: This is the gear that meshes with the rack and is part of the Pinion Housing and Assembly
Helical Rack and Pinion: A helical rack and pinion is a rack and gear set that has the teeth of the rack and pinion cut at an angle. These are more expensive produce because of the extremely fine tolerances required, because several teeth are in simultaneous contact between the two parts. However, helical Rack and Pinion systems have significantly reduced backlash and superior “smooth-feeling” movements, which is why we use them in our FeatherTouch focusers. All of our focusers use brass racks and hardened stainless steel pinions for improved life and performance.
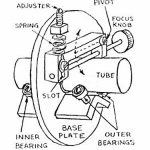
Crayford Focuser Drive: The Crayford focuser drive is a common focusing mechanism in amateur astronomical telescopes. It is named after the Crayford Manor House, Crayford where it was invented by John ‘Jack’ Wall, a member of the astronomical society which meets there. ** Image credit: Astonomics.com with many thanks.
The Crayford is similar in appearance to a Rack and pinion focuser, but has no teeth on either the rack or the pinion. Instead, a round axle is pressed (for example by a spring-loaded or thumbscrew-tightened piece of PTFE plastic) against a flat on the side of the focuser drawtube, relying only on friction to move the drawtube as the axle is turned. This also presses the drawtube against a set of four ball bearings against which it moves smoothly with minimal friction. The pressure exerted on the axle can often be adjusted for smoothest operation, and the drawtube may be locked in position to support heavy eyepieces or cameras.
Subsequently, variations on the Crayford focuser concept have emerged, e.g. a helical Crayford where the drawtube is pressed against four tilted ball bearings, and focusing action is accomplished by rotating the drawtube.
Fine Focus Reduction Unit: This is the unit that will allow very accurate focusing. It is typically a 9:1 planetary reduction assembly that makes focusing extremely precise and easy. We were the original developer of this type of system for telescope use and have been making and improving these for longer than any other manufacturers. These units have undergone continuous improvement and we believe are the best in the industry. It is the key to any of our focusers that allows the user to precisely focus unparalleled with unparalleled accuracy and reliability. We offer three different models that are used with the appropriate focusers. Our smallest being the 2 inch, our intermediate being the 2.7 and our largest being our 3.5 inch. We have had the pleasure of developing the 2.7 inch unit for Astro-Physics and the 3.5 inch unit for TEC and we are now able to offer the 2.7 unit on the 2500 and 3000 Series focusers.
Brake, Internal Brake: This feature allows you to add more drag on the focuser to prevent any downward motion of the focuser and attachments due to gravity. This drag is generated in the Pinion Housing Assembly and is a force applied to the pinion that drives the draw tube back and forth. It can also be locked.
Tube Adapter Ring: This is the part that mates the focuser to the Optical Tube Assemble (OTA) Various options are available and will be offered as the need arises
End Cap: This is the mating part that is screwed into the focuser draw tube. Typically it will be a 2 inch opening using a Compression Ring or a Collet style End Cap. Other options will be considered as the need arises.
Extension: An extension is a tube sections that can be inserted between the OTA and the focuser body thereby increasing the focus range of the focuser.
Focus Travel (also known as Draw Travel): The total distance an inserted eyepiece moves in, and out, of the focuser housing as a result of turning the focus knob on the focuser.
Image-Shift: Image shift occurs on some Schmidt-Cassegrain type telescopes as a result of the mechanism used to obtain course focus. [Contrary to what you may think, the ‘micro focuser’ that is on an SCT (Schmidt-Cassegrain telescope) is called ‘micro’ because of its small physical size even though it is used mostly for coarse focusing.] In a Refractor-style (spyglass) telescopes, the way to achieve focus is to move the eyepiece so that its focal point and focal point of the telescope objective end up in the same place. The micro focuser in an SCT is used to achieve course focus by moving the primary mirror along the baffle tube, to bring the focal point of the telescope to the focal point of the eyepiece. In theory this should work swimmingly, however, because of machining tolerances and material expansion|contraction due to temperature shifts, the mirror may not move in a perfectly aligned motion which will cause the field of view to shift around.
Additionally, something called ‘mirror-slop’ can occur when the mirror meets resistance to movement and binds up, temporarily. You might hear a ‘pop’ when the mirror finally does unbind and settles into it’s final position. In some cases, this can occur hours after you’ve made focus adjustments and can ruin long exposure observations.
Leveling | Collimating Using Your Base
The leveling base is used to collimate (align with the optical axis) the focuser. There are 4 screws that hold the base to the scope and 4 screws that push against the scope. These work to “push-pull” the base to collimate.
Make sure the push screws are backed out. When the base is mounted and all the hold down screws are tight, loosen one hold down screw and tighten that push screw to raise that corner. Then re-tighten that hold down screw. Do not over-tighten. Repeat that until the base/focuser is collimated. This is normally done with a collimating site tube. Normally you only need to do this with one or two corners.
Some trial and error my be necessary to determine which corner(s) needs to be raised. Normally this is a very fine procedure and does not need a lot of adjustment.
Which Focuser…
If your telescope is a Newtonian, where the focuser axis will move in a generally horizontal direction, our standard 2″ focuser should work fine.
If however, the focuser axis of travel is perpendicular, as in Refractor and Schmidt-Cassegrain style telescopes, you may want to consider a focuser with an internal brake.
Also, because the motion of our FeatherTouch Focuser is so smooth and free moving, it may become necessary to increase the friction to hold the eyepiece in place. This might be more of a problem when the direction of the focuser is working more against gravity.
When you choose a focuser, you will need to consider the “focus travel”, also known as “Draw Travel”, which is the total distance an inserted eyepiece moves in, and out, of the focuser housing as a result of turning the focus knob on the focuser.
Some astronomers prefer our draw tube compression ring system as a way of holding the eyepiece in the focuser rather than the standard nylon tipped screws. The compression ring allows for a more secure hold for the eyepiece by utilizing the surface area of the ring. Another option you may wish to consider is the internal brake system which allows the focuser to hold heavier loads. While not usually required on Newtonian scopes, you may wish to consider it if you’ll be using heavy eyepieces and equipment or if the focuser is positioned on your telescope vertical to the ground.
Base plates are mounted on a Newtonian (or Dobsonian) style telescope to hold the focuser in place. Your choice of base plate depends mainly on the surface characteristics of your telescope. Is the place you’re going to mount the base curved or flat? If it’s curved, as in the case of a tube design, you’ll likely need either our Customized Curved Base or our Customized Curved Leveling Base. In both of these cases you will need to supply us with the outside diameter of the tube upon which you’ll be mounting the base. If the place you’ll be mounting the base is flat, you’ll likely choose either our Standard Flat Base or our Standard Flat Leveling Base. Leveling bases, whether flat or curved, assist in the adjustment and collimation of the focuser. FeatherTouch® focusers can be detached from Starlight Instruments’ bases.
Adaptors are used in conjunction with a focuser to allow more flexibility with respect to eyepiece choice. Our adaptors are available in three types: Low Profile, Easy Grip, and Standard. Each one has characteristics that you may wish to consider and all accommodate standard 1 1/4″ diameter eyepieces.
The Starlight FeatherTouch® focusers recommended for Newtonian-style telescopes have draw travel lengths of 1.5″, 2.0″, and 2.5″.
For Refractors larger than 125 mm you may want to consider our 3.5-inch focuser. This focuser was a collaborative design effort between TEC and Starlight Instruments and we feel it is an excellent choice for these telescopes. We feel that this focuser is the best choice for many original or retrofit applications. Some of the reasons why you may want to consider this focuser are as follows:
- It uses a wide fine pitch rack and pinion that is coupled to a 9:1 fine focus reduction assembly similar to our smaller 2 inch focusers giving you the same excellent focusing control as our 2 inch models.
- The drawtube glides on three stainless steel straps that mate with Teflon surfaces to provide very tight yet extremely smooth motion. Using this type of design eliminates the need for grease between the drawtube and housing. A greaseless draw tube prevents the attraction of dirt that may eventually cause problems between these surfaces.
- External adjustment screws can control the tension of the drawtube in the housing thereby allowing the tension to be matched to the equipment that is generally used.
- An additional adjustment/drawtube-locking knob can be used for on the fly adjustment when needed. This same adjustment nut can also be used for additional tightening of the drawtube to prevent any movement of the drawtube relative to the housing during long exposures.
- The drawtube has internally machined baffles that reduce internal reflections.
- Each rack and pinion set is matched and burnished in to provide very smooth engagement between the rack and the pinion.
- The pinions are machined from hardened stainless steel for long life and reliability and the entire pinion block assembly is adjusted and locked in place reducing and virtually eliminating any backlash between the rack and the pinion.
- The large drawtube allows a generous 4.5 inches of travel without vignetting at f5 or larger.
- The entire focuser can be rotated relative to the telescope tube thereby making orientation of the focus knobs convenient. This also allows the positioning of objects and the location of guide stars much easier. The focuser body is located in a tube adapter ring and locked in place with the focuser-locking ring. When this ring is turned and loosened slightly the entire assembly can be rotated. Once the desired position is reached the focuser locking ring is tightened thereby locking the entire assembly.
- The end of the draw tube is fitted with a 2 inch collet attachment for 2 inch eyepieces. This collet attachment locks onto the eyepieces using a plastic sleeve that is compressed This eliminates the use of thumb screw and always assures that the eyepiece is perfectly aligned.
For smaller refractors we would recommend either our 2″ modified Crayford-style with either the 2″ or 2.5″ draw tube.
For Schmidt-Cassegrain telescopes, the 2″ focuser with either the 0.8″, or the 1.5″ draw tube will work.
If the Schmidt-Cassegrain uses a fork-type mount, the 0.8″ draw tube travel option, combined with the “shorty adapter” will provide clearance between the focuser and the fork-mounting in most cases.
The 0.8″ draw tube travel focuser with the shorty adapter was specifically designed for the Meade LX200 telescope so that when the focuser passes through the mount, enough clearance will prevent any potential crashes in the go-to mode.
Because the motion of the FeatherTouch® Focuser is so smooth and free moving, it may become necessary to increase the amount of friction between the pinion and the draw tube in order to hold the eyepiece in place.
How Important is Focusing?
Next to having great optics, eyepieces and excellent collimation, focusing, in our opinion, is equally as important. In general, focusing needs to be precise and allow positioning of the eyepiece or imaging equipment within .002 inches.
There should be no backlash in the focuser and the focuser should be very free to move. Since our focuser uses a precision 10:1 planetary reduction assembly, this makes focusing extremely easy. Positioning of the draw tube and eyepiece within .0005 inches can easily be achieved. Not only is the positioning of the eyepiece extremely precise but because our focusers are so smooth, the slightest touch on our 10:1 reduction fine focus knob will allow focusing without shaking of the telescope tube.
Why should I consider a FeatherTouch Focuser?
Not only should the focuser provide precision positioning and be easy to use, it should also permit effortless focusing without shaking the telescope. Our 10:1 reduction ratio and the precision of the design make this possible. Since there is virtually “ZERO BACKLASH”, focusing at even very high power is done with ease.
Even though our focusers are slightly more expensive keep in mind that we have designed and built this unit to last a lifetime. You have probably invested much more in your eyepieces and use each of these eyepieces with the focuser, so why not buy a FeatherTouch® Focuser that will permit the full performance of your telescope?
What is the Brake Option, and do I need it?
The brake option was designed to allow users of refractor type applications the ability to gradually increase the friction between the pinion and the drawtube. In our non brake version design we use a thumb screw to lock the pinion shaft and to also increase the amount of drag on the drawtube.
This system however has very little range when trying to control the drag.
The brake consists of an internal leveraged leaf spring that is tightened with a thumbscrew, thereby allowing a much greater control of the drag. The same thumbscrew is also used to lock the pinion shaft.
How much weight can the 2″ FeatherTouch Focuser handle?
For refractor applications the same rules apply and generally 5 lbs. of weight is not a problem although this also depends on the length of the draw and where the weight is applied. Since the longer draw tubes increase the leverage and the amount of force on the bearings the weight and the leverage need to be considered in the overall equation.
We have designed our focuser to comfortably handle at 5 lbs. with the 2.5 inch (our longest drawtube) and have not heard of any problems from our customers. Since even the heaviest eyepieces and most imaging equipment does not weigh more than this, the weight limit should not be a problem in most applications. If you have a particular applications where this may be a concern please do not hesitate to consult us on your application.
What length draw tube do I need?
This depends largely on the your eyepieces and the equipment that will be used on the focuser. In general the 2 inch focuser draw tube seems to work well for most applications. One of the best ways of determining the needed travel is to measure the position of each of your eyepieces on an existing telescope focuser relative to a fixed location on the existing focuser. Measure the location for each eyepiece and combinations of eyepieces with a scale or other suitable means. From these locations determine the difference between the minimum to maximum distance. The resulting distance will be the minimum travel you would need.
Keep in mind if a 35 mm camera or CCD camera is used you will need to make sure that the focal plane can also be reached. It is also important to keep in mind that our focuser draw tube will retract .2 inches into the housing of the focuser body thereby preventing the use of the extra .2 inches of travel. This extra in-travel was originally intended to permit improved performance for Newtonian applications where position of the eyepiece relative to the secondary plays an important role in the size of the secondary and the overall performance of the telescope. Therefore this extra .2 inches of in-travel will only work for 1.25 eyepieces and will interfere with eyepieces or equipment that will not clear the bearings and housing.
Intro to Telescopes, 101
There are three primary types of optical telescope: Reflectors (Catoptrics) which use mirrors, Refractors (Dioptrics) which use lenses, and Combined Lens-Mirror Systems (Catadioptrics) which use lenses and mirrors in combination.
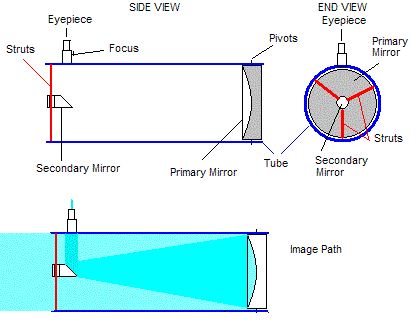
The most popular telescope design is the Newtonian Reflector.
The Newtonian has the advantage of being a simple design that allows for maximum size for the minimum expense. And since the design employs a single front surface mirror as its objective it means (for the person creating the optical elements) that there is only one surface that has to be ground and polished, as opposed to three for the Maksutov and four for the refractor and the Schmidt-Cassegrain. A properly designed and built Newtonian is a high performance instrument, fully capable of matching the performance of other designs, a case where inexpensive does not mean inferior. Typically a Newtonian Telescope of 6″ or 8″ (15 or 20cm) aperture is considered a good starter project. Some widely available plans found on the Internet include instructions for building 6″, 8″, and 10″ Newtonians.
A type of Newtonian, the Dobsonian, is a design that has become popular amongst amateur astronomers because it results in an extremely simple and rugged large-aperture instrument at low cost. The term “Dobsonian” or “Dob” refers to any telescope with an alt-azimuth mount and a Newtonian telescope tube assembly that feature several innovations made popular by John Dobson.
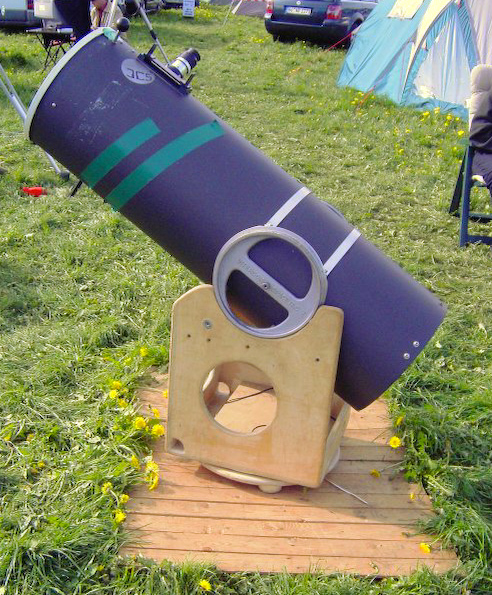
The ‘classic hard tube’ or first-generation Dobsonian is the type that was first popularized by John Dobson and the San Francisco Sidewalk Astronomers in the late 1960’s as part of their mission to bring astronomy to the masses.
The basic idea driving the original design is to make large aperture telescopes affordable, easy to make, and portable. It is a combined concept that allows the builder with minimal skill to make an extremely large telescope out of common items found in any hardware store or scrap yard.
Dobsonian style telescopes are optimized for visual astronomy (deep sky observing), i.e. visually observing star clusters, nebulae, and galaxies that require a large objective mirror with a lot of “light gathering” capability. Since deep sky observing requires the observer to travel out to dark locations away from city lights the design had to be more compact, portable, and rugged than the standard large Newtonian telescope.
Telescopes built on the very popular Dobsonian design employ a variant of the alt-azimuth mount due to its ease of construction.
An Altazimuth or alt-azimuth mount is a simple mount used for moving a telescope, or any other object, along two perpendicular axes of motion–one vertical and one horizontal. The vertical movement is known as the altitude, while the horizontal motion is called the azimuth.
The biggest advantage of alt-azimuth mounts is their simplicity in both manufacture and use. They are often used for beginner telescopes, or for spotting scopes, but are still widely in use for more advanced instruments. In the latter case, advanced electronics and motors are sometimes attached to compensate for the restrictions of the mount’s simplicity.
In astronomy, alt-azimuth mounts were, for a time, surpassed in popularity by the more complex equatorial mount. The latter is more naturally suited for tracking astronomical objects in the night sky as the Earth spins on its axis, since its polar alignment means that only one axis need be adjusted rather than the two of an alt-azimuth mount. Being able to track such objects reliably is particularly important for astrophotography, as well as more advanced amateur astronomy, both of which became more accessible when equatorial mounts became affordable.
In recent decades, alt-azimuth mounts have once again become very popular for astronomical telescopes:
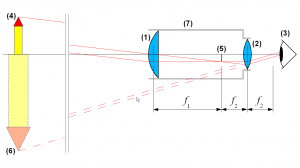
A refracting or Refractor telescope is a dioptric telescope that uses a lens as its objective to form an image. The refracting telescope design was originally used in spy glasses and astronomical telescopes but is also used in other devices such as binoculars and long or telephoto camera lenses. Refractors were the earliest type of optical telescope. All refracting telescopes use the same principles. The combination of an objective lenses (1) and some type of eyepiece (2) is used to gathered more light than the human eye could collect on its own, focus it (5), and present the viewer with a brighter, clearer, and magnified virtual image.
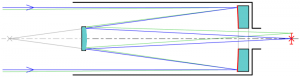
Catadioptric telescopes are designs that combine specifically shaped mirrors and lenses to allow very fast focal ratios (when used at the prime focus), while controlling coma and astigmatism. The “Classic” Cassegrain has a parabolic primary mirror, and a hyperbolic secondary mirror that reflects the light back down through a hole in the primary. Folding the optics makes this a compact design. On smaller telescopes, and camera lenses, the secondary is often mounted on an optically flat, optically clear glass plate that closes the telescope tube. This support eliminates the “star-shaped” diffraction effects caused by a straight-vaned support spider. The closed tube stays clean, and the primary is protected, at the cost of some loss of light-gathering power.
It makes use of the special properties of parabolic and hyperbolic reflectors. A concave parabolic reflector will reflect all incoming light rays parallel to its axis of symmetry to a single point, the focus. A convex hyperbolic reflector has two foci and will reflect all light rays directed at one of its two foci towards its other focus. The mirrors in this type of telescope are designed and positioned so that they share one focus and so that the second focus of the hyperbolic mirror will be at the same point at which the image is to be observed, usually just outside the eyepiece. The parabolic mirror reflects parallel light rays entering the telescope to its focus, which is also the focus of the hyperbolic mirror. The hyperbolic mirror then reflects those light rays to its other focus, where the image is observed.
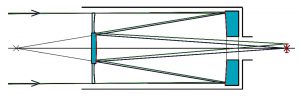
The Schmidt-Cassegrain [SCT] is a catadioptric telescope. It combines a folded optical path with a corrector plate to make a compact astronomical instrument. This design is very popular with consumer telescope manufacturers because it combines easy to manufacture spherical optical surfaces to create an instrument with the long focal length of a refracting telescope with the lower cost per aperture of a reflecting telescope. The compact design makes it very portable for its given aperture, which adds to its marketability. Their high f-ratio means they are not a wide field telescope like their Schmidt camera predecessor but they are good for more narrow field deep sky and planetary viewing.
While there are many variations, (both mirrors spherical, both mirrors aspherical, or one of each) they can be divided into two principal design forms: compact and non-compact. In the compact form, the corrector plate is located at or near the focus of the primary mirror. In the non-compact, the corrector plate remains at or near the center of curvature (twice the focal length) of the primary mirror. Typical examples of the compact design are Celestron and Meade commercial instruments, combining a fast primary mirror and a small, strongly curved secondary. This yields a very short tube length, at the expense of field curvature. Most compact designs from Meade and Celestron have a primary mirror with a focal ratio of f/2 and a secondary with a negative focal ratio of f/5 yielding a system focal ratio of f/10. One notable exception is the Celestron C-9.25, which has a primary focal ratio of f/2.3 and a secondary focal ratio of f/4.3, the result being a slightly flatter field and a slightly longer tube aspect ratio than most other compact designs.
Non-compact designs keep the corrector at the center of curvature of the primary mirror. One very well-corrected design example would be the concentric (or monocentric) Schmidt-Cassegrain, where all the mirror surfaces and the focal surface are concentric to a single point: the center of curvature of the primary. Optically, non-compact designs often yield better aberration correction and a flatter field than a compact design, but at the expense of longer tube length
Drawbacks to the Schmidt-Cassegrain design include image shifting during course focus, and its susceptibility to temperature changes. Since course focusing is usually achieved by moving the primary mirror, the image may shift unless everything has been machined to extremely high tolerances. Additionally, binding can occur, resulting in both image-shift and mirror-slop when the mirror unbinds.
** Definitions, in quite a few cases, were obtained from Wikipedia, the free, online encyclopedia. If you are new to astronomy, it is an excellent resource with great explanations and lots of helpful examples.